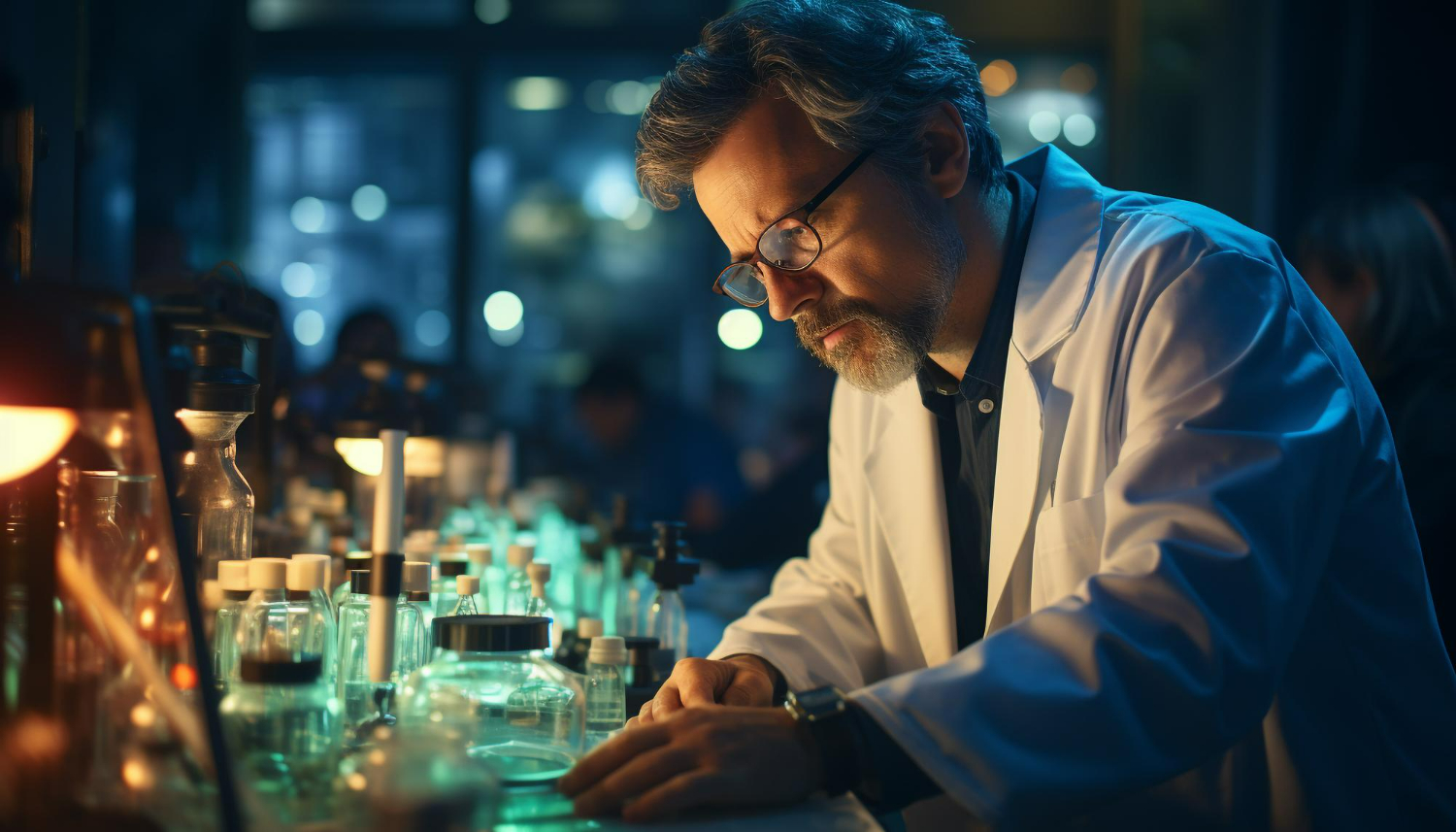
Before recently, the science of gene editing—which earned scientists Jennifer Doudna and Emmanuelle Charpentier the 2020 Nobel Prize in Chemistry—seemed more like science fiction than medicine; where Individuals are cured of disease by using lab-created molecular scissors to cut out problematic DNA sections. Interestingly, we are on the verge of seeing regulators approving the very first treatment using gene-editing technology to combat rare inherited blood disorders.
Technologies for gene editing have become extremely useful for deciphering and modifying genetic data. These techniques were initially mostly used in genetic therapy and the treatment of diseases, but they are now also being used in drug discovery. By combining expertise in genetics, bioprinting, and pharmaceuticals, we can unlock new frontiers in medicine.
The global genome editing market size was valued at USD 6.39 billion in 2022 and is expected to grow at a compound annual growth rate (CAGR) of 17.8% from 2023 to 2030.
This article entails the utilization of genome editing tools and technology and their impact across the drug discovery industry.
The Genesis of Gene Editing in Drug Discovery
Exact gene editing enables researchers to build more realistic disease models for drug development. The development of personalized medicine is facilitated and potential drug targets are better identified as a result of the increased precision.
By modifying the sequence or expression of genes through gene editing, researchers can create a variety of assays to pinpoint disease targets and evaluate the effectiveness of treatments.
Knockouts, knockins, or nucleotide exchanges are made easier by subsequent cellular repair. Because these types of modifications are made endogenously, scientists can study the subsequent changes to mRNA and protein at native, physiologically relevant levels.
Gene editing tools in practice: Advantages and Limitations
The transcription activator-like effector nucleases (TALENs), zinc finger nucleases (ZFNs), and the clustered regularly interspaced short palindromic repeats-Cas system (CRISPR-Cas) are the most widely used molecular scissors.
Though TALENs and ZFNs are practical technologies, their design and structure are more complex and require specialized protein design, making them more costly and time-consuming than CRISPR. In addition, their accuracy and specificity are less than those of CRISPR/Cas9.
CRISPR systems are significantly easier to create and operate because they only require a nuclease (typically CRISPR-associated protein 9, or Cas9) and a guide RNA (gRNA). The use of CRISPR has started as a revolution in the field of genome editing and has now become increasingly normality and a widely used technology for screening applications in Drug Discovery, especially in functional genomics.
Drug discovery workflow: Engineering novel therapeutics with CRISPR
The drug discovery process often starts with basic scientific research and involves many steps before new therapeutics are approved for clinical use. Most preclinical processes share several common steps, including target identification and validation, high-throughput compound screening, hit validation, and lead drug candidate optimization. Below is a discussion of each of these steps and how CRISPR is facilitating advancement through them.
Finding the Target through CRISPR
Finding a drug target—a gene, an RNA transcript, or a protein—associated with a desired disease is the first step in the drug discovery process. Large numbers of candidate genes can be systematically knocked out, inhibited, or activated using CRISPR-based high-throughput screens. Potential therapeutic targets can be identified by perturbations that worsen or impede an illness.
By enabling gene knockouts or protein overexpression in cell lines, CRISPR may help these processes. A screening campaign to find possible therapeutic candidates is started if a causal relationship between a target and disease is established, and if the target is druggable.
CRISPR in Compound screening
CRISPR has improved cell-based screens by enabling scientists to more accurately generate cell lines with mutations relevant to the disease of interest. Multiple mutations can be induced simultaneously with CRISPR to better mimic disease genotypes, according to a study published in the Computational and Structural Biotechnology Journal.
These preliminary screens can precisely rule out ineffective compounds and pinpoint the most effective ones early in the drug discovery process, saving time and money, thanks to improved cellular models. Hit molecules that make it past this screening stage are examined in greater detail in the following pipeline stage.
Hit validation: CRISPR doing the Job
A range of cell-based tests are used to validate hits found by the first screens. This stage of preclinical development has been significantly impacted by CRISPR because it has made it easier to create models that faithfully represent diseases.
Scientists can create primary cells, stem cells, and organoids with the right cellular and genetic backgrounds instead of being restricted to immortalized cell lines. Human induced pluripotent stem cells, or hiPSCs, can be used to create almost any type of cell, according to a study published in the journal Development. It is simple to create isogenic cell lines from these stem cells, and with careful genome engineering, it is possible to replicate the genetic variation linked to a disease.
Lead identification and optimization
The number of candidates is reduced to a select few leads by the validation of hits. The remaining substances are currently being tested for safety and optimization.
Because CRISPR has made it possible to create cellular and animal models that are used to evaluate the safety and efficacy of candidates, this phase of research has advanced more quickly. For example, instead of crossing single-mutant animals, it is now possible to generate mouse models with multiple mutations in a single step by directly editing zygotes. Furthermore, a greater variety of animal models will probably be able to be created thanks to CRISPR.
Clinical trials and FDA approval
Clinical trials are the next step for the few surviving candidate compounds after optimization and safety testing. The FDA never considers approving a drug candidate until it is both safe and effective in human trials.
Gene Editing in Bioprinting: Building the Future of Drug Testing
While gene editing refines drug target identification, bioprinting takes drug testing to the next level. A study, published in the Annals of Translational Medicine, suggests that gene editing can help bioprinting in several ways. These include improving the compatibility and functionality of the bioprinted tissues and organs, producing personalized and customized products, and adding new features and functions to the bioprinted structures.
Preclinical testing is revolutionized by this development, which provides a more realistic picture of how medications interact with human tissues.
Ethical and social issues
Gene editing technologies pose many ethical and social issues that need to be addressed and regulated. Some of these issues include
- Safety and efficacy of gene editing and bioprinting
- Potential for unintended consequences and off-target effects
- Possible misuse and abuse of gene editing and bioprinting for malicious or unethical purposes
- Impact of gene editing and bioprinting on biodiversity and ecosystems
- Respect for the rights and dignity of living things that are the subject of bioprinting and gene editing, as well as the equitable sharing of the advantages and disadvantages of these technologies among various stakeholders and groups.
In addition to the creation of suitable guidelines, standards, and policies, these issues necessitate serious thought and consultation across numerous disciplines, sectors, and communities.
To conclude
Gene editing shows a great deal of promise for pharmaceutical development. Collaboration between academic institutions, pharmaceutical companies, and technology innovators is critical for the future of drug discovery.
The transition from Big Pharma to bioprinting signifies a change in the direction of a more effective and patient-centered approach to drug discovery, one that has the power to fundamentally alter how we perceive, diagnose, and treat illnesses.
Furthermore, AI makes it possible to customize treatments based on genetic profiles in conjunction with genome editing and precision medicine. AI examines the genetic information of patients to find mutations, variations, and biomarkers linked to various illnesses.
In this world of healthcare advancement, Codewave EIT’s AI solutions insightfully enable the diagnosis of complex problems, the prediction of possible solutions with a probability of success, discovery of potential new drugs.
Codewave’s intervention includes:
- Diagnosing & identifying opportunities where AI can have maximum impact
- Designing & architecting AI-aided solutions
- Designing & Developing a rapid prototype to test the solution
- Designing & Developing the solution, for full-scale deployment
- Reviewing outcomes regularly & continuously optimizing results
To understand the impact of innovative technologies in gene editing and drug discovery you can talk to our team. We are here to bring healthcare to the next level for the betterment of Mankind.